The article provides information on the current (for 2013) results of the search for supersymmetry - one of several speculative ideas about what may be outside of known particles and interactions. Supersymmetry is one of the options (the most popular and perhaps the most criticized - but not the only one) of what can solve the so-called “
naturalness ” problem, which is closely related to the “
gauge hierarchy problem ”. Why is gravity so weaker than other interactions? Why is the mass of the Higgs particle so small compared to the mass of the smallest possible black hole?
In mid-2011, when the Large Hadron Collider (BAC) was still young, my colleague John Conway
announced in his blog that supersymmetry (specifically, supersymmetry as a solution to the problem of naturalness, which I will call “natural supersymmetry”, the EU)) In essence, it was rejected by data obtained in the ATLAS and CMS experiments at LHC. A quick glance and a couple of minutes was enough to understand that this statement was erroneous - and this demonstrates the fact that people continue to search for signs of the EU until now. Why is it so difficult to reject the EU? Because this topic has a huge number of options - incredibly many options for supersymmetry that can solve the riddle of naturalness. To eliminate them all, it will take a lot of work! Much more data than was collected at the LHC in a few months.
By the middle of 2012, having received five times more data and having done much more work on sorting them, we realized that the situation was a little more complicated. This time it took a little more time, about a few hours, to understand that the results with ATLAS and CMS did not reject the EU. There were two difficulties. The first is the results of a very important measurement from the LHCb experiment (although the experimenters managed to confuse the public twice, mistakenly stating that they excluded the possibility of the EU, or at least “sent it to a hospital bed” - one of the most meaningless statements in the field of physics, which I have heard). The second is the discovery of a Higgs particle with a mass of 126 GeV, easy enough to satisfy the EU, but too heavy to fit its simplest variants. And yet it was too early to make any specific statements about the EU or anything else.
What is happening today [2013]? What took a couple of minutes in 2011, and a few hours in 2012, in 2013 required six months of hard work. The data collected in 2012 on ATLAS and CMS turned out to be much larger in volume, and experimenters had to spend much more time trying to get through them. After all the effort spent, it became possible to understand which variants of supersymmetry are excluded and which are not. We are finally in a position that allows us to begin to draw important, though incomplete, conclusions about the natural variants of supersymmetry.
As I said, supersymmetry has a staggering number of options, each of which makes slightly different predictions regarding LHC experiments. In principle, it is possible, using existing data from ATLAS and CMS, to make certain statements about the EU that are not specific to any particular subclass of supersymmetry variants - but can statements be made that apply to all (or to a very large class) EU theories? My colleagues and I answer this question in
our scientific work .
We have shown that the answer will be positive. This “yes” is a bit limited, since there are several small logical loopholes, but I will emphasize - small ones. Compared with 2012, this is a major improvement, because a truck could be carried through those loopholes. Here are our arguments:
First, we assume that we are dealing with a natural variant of supersymmetry, which requires that
Higgsino (theoretical supergroups of Higgs particles — supersymmetry requires five kinds of Higgs particles) have a mass of not more than 400 GeV / s
2 . And this is a fairly conservative requirement - most of the EU options require that the particles be much lighter.
Secondly, we assume that gluino (gluon superpartners) are available to us in the following sense: their mass does not exceed 1400 GeV / s
2 , and this is enough for us to get several such particles during data collection in 2011-2012 .
Then we note the following: if, and when, gluinoes are produced at the LHC in proton collisions, then in almost all EU models with available gluinoes one or more of the following phenomena will occur:
- Missing transverse momentum. A vivid sign that both observable and unobservable particles are created in collisions, and the observables obviously rebound from those that we cannot see.
- Upper quarks and antiquarks: fairly heavy particles (the upper quark mass is 175 GeV / s 2 ), often decaying into an electron or muon, a neutrino (unobservable) and a lower quark (or their antiparticles).
- A large number of high-energy elementary particles: quarks, antiquarks, gluons, leptons, antileptons or photons. A typical amount is from 3 to 10 elementary particles per gluino, and therefore from 6 to 20 particles in a proton-proton collision.
Finally, we point out that the search for all these experimental features was carried out efficiently and practically without assumptions, both on ATLAS and CMS. The search for the first two signs is so precise that it is almost impossible to miss the EC model, in which gluino splits into the top quark and a missing transverse momentum occurs if the gluino mass does not exceed 1000 GeV / s
2 , and sometimes can reach up to 1200 GeV / s
2 . If during the decay of gluino several upper quarks arise and there is practically no missing transverse momentum, but many quarks, antiquarks and gluons appear, then the limits on the gluino mass become weaker - perhaps around 800 GeV / c
2 , but usually there are in the region of 1000 GeV / c
2 . We have also indicated how to improve the search for gluino in this last category.
This set of observations excludes most of the EU variants, in which the mass of gluino is in the available area, up to or around 1000 GeV / s
2 . Only variants with more severe gluino, or with the decay of gluino, in which none of the three above-mentioned signs are observed, or with unnaturally heavy Higgsino, do not fall here. The result is summarized in the figure below. What is important, unlike earlier searches for supersymmetry, based on three key assumptions of the most popular versions of supersymmetry:
- In any process, the number of superpartners can only change to an even number.
- The lightest superpartner (which, as follows from item 1, is stable) is the superpartner of a particle known to us (and, therefore, in order to avoid conflicts with the available data, it is unobservable neutralino or snaytrino).
- Superpartners, on which strong nuclear interaction acts, are much heavier than other superpartners of particles known to us.
our results are applicable, even if we reject any or all of these assumptions. We also do not assume that supersymmetry is “minimal” - that is, that we have to discover only superpartners of particles already known to us (and additional Higgs particles required by supersymmetry).
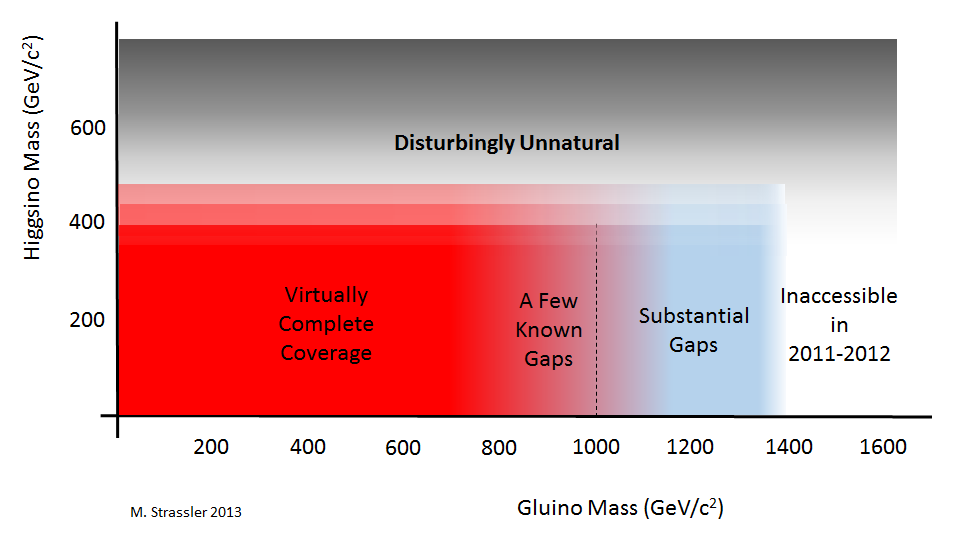
Vertically - the Higgsino mass, horizontally - Gluino. The top of the chart is unpleasantly unnatural results. Below to the left is almost complete coverage, then, from 800 to 1000 GeV / s
2 - several known gaps, then large gaps, and then, from 1400 GeV / c
2 - uncharted territory.
And how can one characterize the search for natural supersymmetry in this connection? You could say they are 3/4 complete. For EU variants without gluino, which could have been obtained in 2011–12, there have been many searches for other super-partner particles — but, as
shown by my colleagues Jared Evans and Yevgeny Katz, this cannot be called full coverage. For example, there have been many searches for the upper squawks, superpartners for the upper quarks, but each of them had to make certain assumptions about how the upper squars break up. And for such a decay, there are opportunities beyond the current search methods. The same is true for Higgsino and other similar superpartner particles.
Eliminating the EU with almost complete certainty and very small loopholes will not work until the LHC has worked for another couple of years with proton collisions at energies of 13 TeV - and this work will begin only in 2015 [From 2015 to 2017, the LHC actually worked with This estimated power is currently processing the results / approx. trans.]. By 2017, we will have to get data that excludes almost all EU variants with a mass of gluino up to 1600-1800 GeV / s
2 (if we don’t open one, of course). By that time, the limitations on the upper squars and Higgsino will also be much stronger, and this will leave very little room for supersymmetry.
I want to mention a couple of loopholes in our logic. The largest of them is our assumption that during the decay of gluino there are no new long-lived particles or other strange phenomena. Such capabilities would require a completely different set of strategies, and it is difficult to study them without knowing the details of how the detectors measure long-lived particles — this topic is quite complex. For some types of long-lived particles, the available searches are very well suited, for others no searches have been undertaken at all - therefore the coverage of this topic is very fragmentary. In addition, we assumed that the masses of gluino, Higgsino, and all other superpartner particles of small mass are not located in a very narrow gap several tens of GeV / s
2 wide. For such a case, special measures will be required, except for those undertaken by us - however, it is not yet clear whether such a development is possible within the EU. Finally, in principle, one can imagine such a complex decay of gluino that it will confuse any modern search methods. Whether such decays can exist is a matter of a separate theoretical study. Perhaps there are other loopholes, but we consider them quite small.
What else is important to understand for those who are not experts is when experimenters with ATLAS or CMS say that “we have completed the search for X”, where X is a particle of some kind or a phenomenon or idea, this does not mean that this search it was necessarily useless for Y, where Y is very different from X. Experimenters are looking for supersymmetry, not only because it can be found, but also because the strategies they use can reveal something else to us. Conversely, sometimes searches for something not related to supersymmetry are useful for searching for certain supersymmetry variants.
Simply put, even if you sincerely believe that X does not exist, this does not mean that you need to assume that the search for X is a complete loss of time. No need to criticize the experimenters for the lost “in search of supersymmetry” or “extra dimensions” or something else. The same searches are useful and necessary for the discovery or elimination of many other theories. For example, in our work, we showed that certain searches for additional spatial dimensions (more precisely, searches for microscopic, instantly evaporating black holes) are one of the most fruitful ways to eliminate gluino, with the decay of which many elementary particles appear.
As a result, I would like to think that our results represent a step forward towards understanding that ATLAS and CMS inform us about particle physics, and how we will need to conduct searches in the future. One possible lesson will be that in many situations a small number of broad and comprehensive searches for a general phenomenon are more effective than a large number of highly optimized and very narrow searches for a completely specific phenomenon. In the latter case, many more holes remain, and if they do not make discoveries, they turn out to be less useful than the first case, in terms of drawing up general and firm conclusions about how the world works.