The productivity of workstations and processor power increase with each new generation. Accordingly, the cooling possibilities must change. For example, the 10-core Intel Xeon E5-2687W (Haswell) v3i thermal pack increased from 150 to 160 W compared to its 8-core predecessor, Intel Xeon E5-2687W v2ii (Ivy Bridge). Currently this workstation is equipped with 22-core processors. The problem is aggravated by the use of hardware platforms and enclosures of the same size: engineering solutions for cooling in a compact form factor are becoming crucial for the performance and acoustic characteristics of the product.
A fundamentally new level of speed is provided by two most modern processors of the Intel Xeon E5-2600 v4 series with support for up to 22 cores each. The
Precision 7910 Workstation in the Tower package is a solution for loads that require large computational resources (visualization tasks of complex processes, tools for
modeling and analyzing large amounts of data). Dell's liquid cooling system reduces noise by 38%.
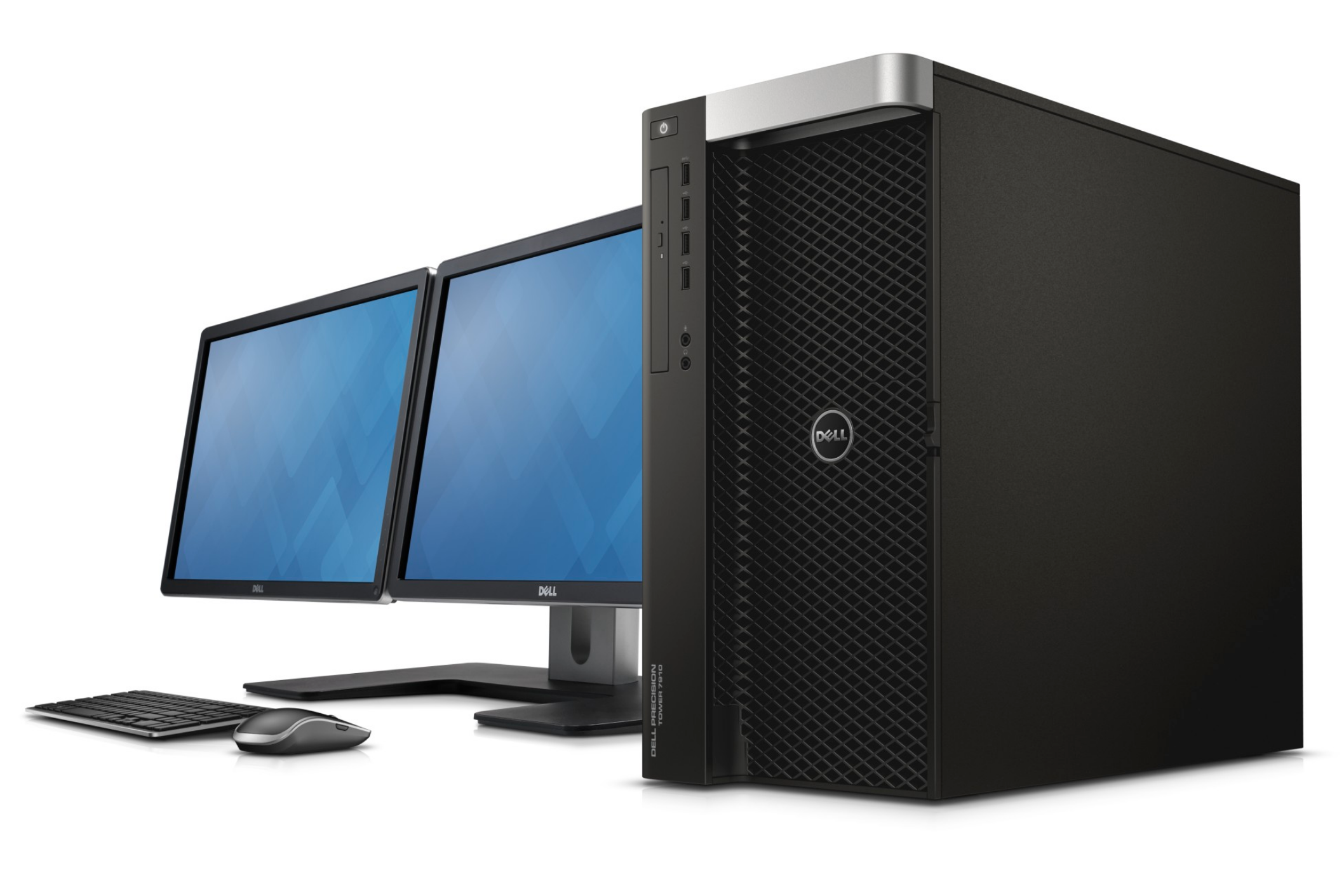
With increasing requirements for the computational process, it becomes increasingly difficult to organize the cooling of increasing capacities. To solve this problem, Dell has developed an innovative system with liquid cooling: the potential of heat removal in it has tripled compared to traditional solutions, and the noise level is optimized.
Air cooling using heat pipes and aluminum radiators is reliable enough, but requires significant air flow. For him, in turn, need fans with high speed. However, they create strong noises.
To reduce the fan rotation speed and, accordingly, the noise level, it is necessary to optimize the performance and minimize the cooling requirements. The Dell solution uses innovative side-blower liquid cooling, which redistributes unused chassis volume to maximize cooler performance and minimize noise.
Dell Precision Tower 7910 Liquid Cooling WorkstationLiquid-Cooled Workstation Physics
To appreciate the benefits of liquid cooling, it is important to understand the basics of what constitutes a highly efficient solution in this area. Any air-cooled or liquid-cooled radiator is characterized by a basic property called thermal resistance [R]. It is measured in degrees per watt and is a function of device temperature and power:

Legend:
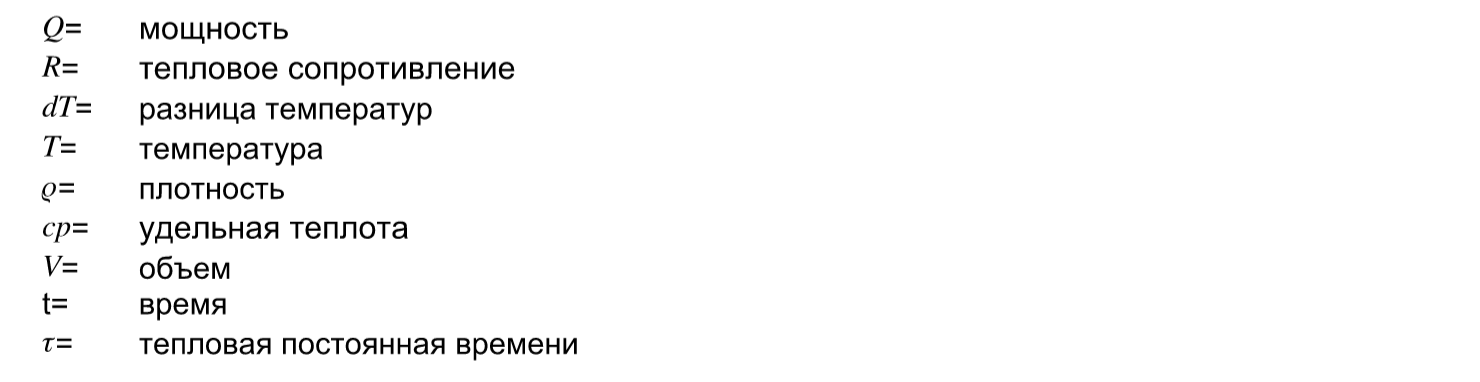
For our particular case, Tj refers to the temperature of the CPU, and Tamb is the local ambient temperature (at the entrance to the cooling radiator). A good analogy is the water flowing into the sink. If the water entering the sink from the tap is considered as the power supplied, then the resistance value R is determined by how tightly the cork is closed (minimally open — high R values, fully open — small R values), and delta T is related to the water level in the sink .
How temperature is related to thermal resistance. The water in the sink represents energy.The second key relation for any cooling device is determined by the amount of energy in the material of the heat sink per unit time. This transition is expressed in the following equation:

If the left side of the equation represents the relationship between the density, the volume of the radiator and its heat capacity, then the right side is the exchange of energy flowing into and out of the radiator. Here again you can draw an analogy with large and small shells. With the same amount of fluid falling into each of them (power), the water level (temperature) in a larger sink will grow more slowly.
How temperature is related to heat capacity.From equations 2 and 3, you can determine the difference in efficiency between any two cooling solutions and see how their performance varies with time. As follows from equation 3, for materials with a high heat capacity (cf), the temperature change with time (dT / dt) will be small for the same power supplied. In addition, from equation 2 we know that the absolute increase in temperature is directly related to thermal resistance.
From these two fundamental ratios, it can be seen that the key to maximum cooling efficiency is low thermal resistance and high heat capacity. Next, we look at these two properties and how they relate to the measured characteristics of the Dell Precision Tower 7910.
Characterization of a liquid cooled workstation
Thus, the cooling efficiency depends on both the heat capacity [cp] and the thermal resistance [R]. This can be used to study the effect of liquid cooling on a Dell workstation. Since the heat capacity of any cooling solution is of key importance in terms of the heating rate of the system, in order to understand how fast the fans should work, it is important to take into account the materials used in the cooling technology. The following table illustrates the heat capacity of various materials - from the most efficient to the least:

The table confirms what most people already know: water has a significantly higher heat capacity than almost all other materials. In fact, with the same mass, the water will heat up to four times longer than aluminum, and more than ten times longer than copper.
Since efficiency depends not only on heat capacity [cp], we also need to understand the ratio of thermal resistance and overall performance. In most coolers, the resistance [R] is determined mainly by the surface area and air flow rate over the cooling radiator. Simply put, the larger the cooler's surface area and air velocity, the better the heat dissipation will be.
Dell used both of these phenomena in its Liquid Cooled Tower 7910 design to maximize efficiency and minimize noise. For this particular study, we used a configuration that includes two 160W Xeon E5-2680 V3 processors, an NVidia K6000 card, 2 x 8 GB Hynix memory modules, and a SATA hard disk with a capacity of 1500 GB.

To maximize the heat storage potential and minimize thermal resistance, 110 x 92 mm radiators with large internal tanks were used. This allowed engineers to increase the volume of fluid in the cooling system, as well as expand the surface area in the available volume of the body.
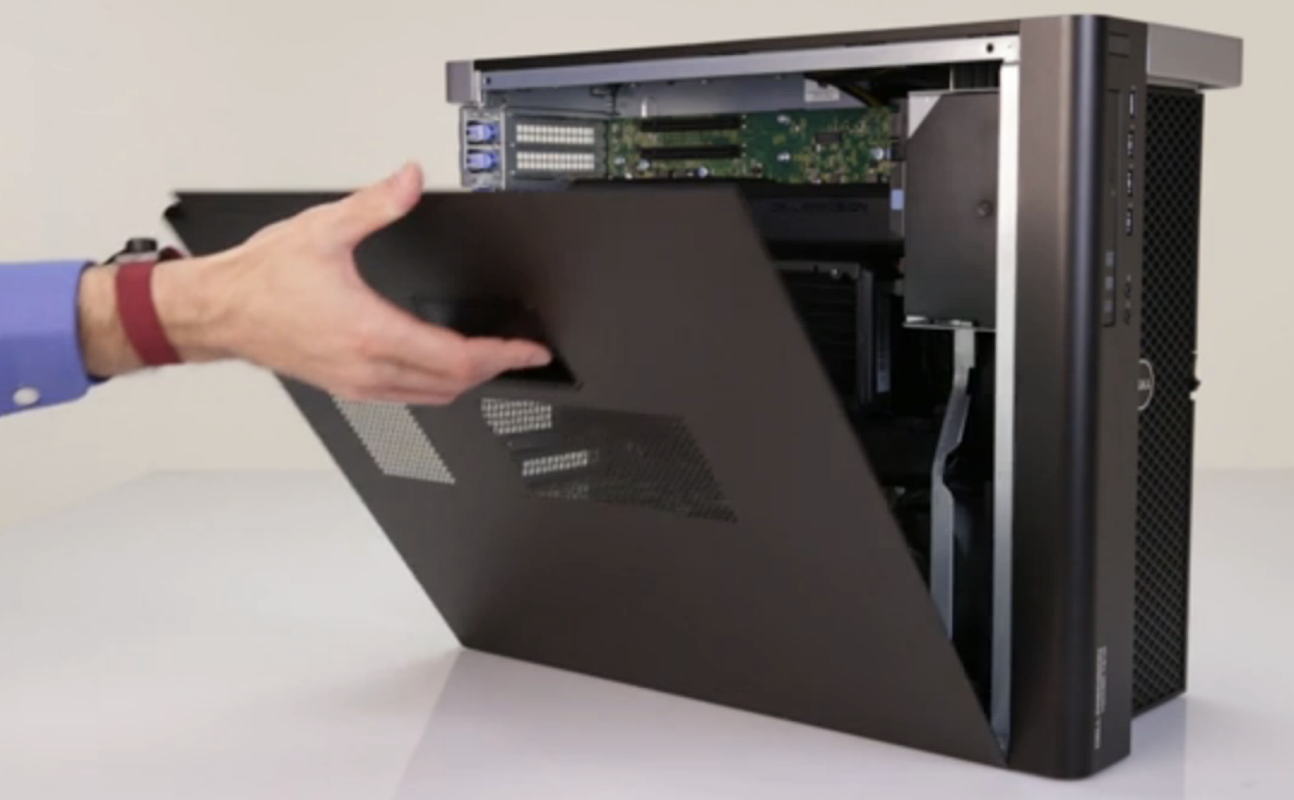
In addition, an innovative approach to the distribution of air flow in the system was used. The figure below illustrates the layout of the liquid-cooled solution in the Precision Tower 7910.
Blowing air through the side wall of the housing.As you can see, the cooling air enters from the front of the system and is supplied to both processors. The air flow passes through the radiator, where energy is exchanged, and all the heat absorbed from the CPU is removed from the liquid and discharged into the room outside the system. This “side exhaust” strategy allows you to use the shortest way to remove the heat produced and more efficiently place the components of the cooling subsystem, as well as reduce the speed of rotation of the fans (because they do not need to drive air along the entire length of the case).
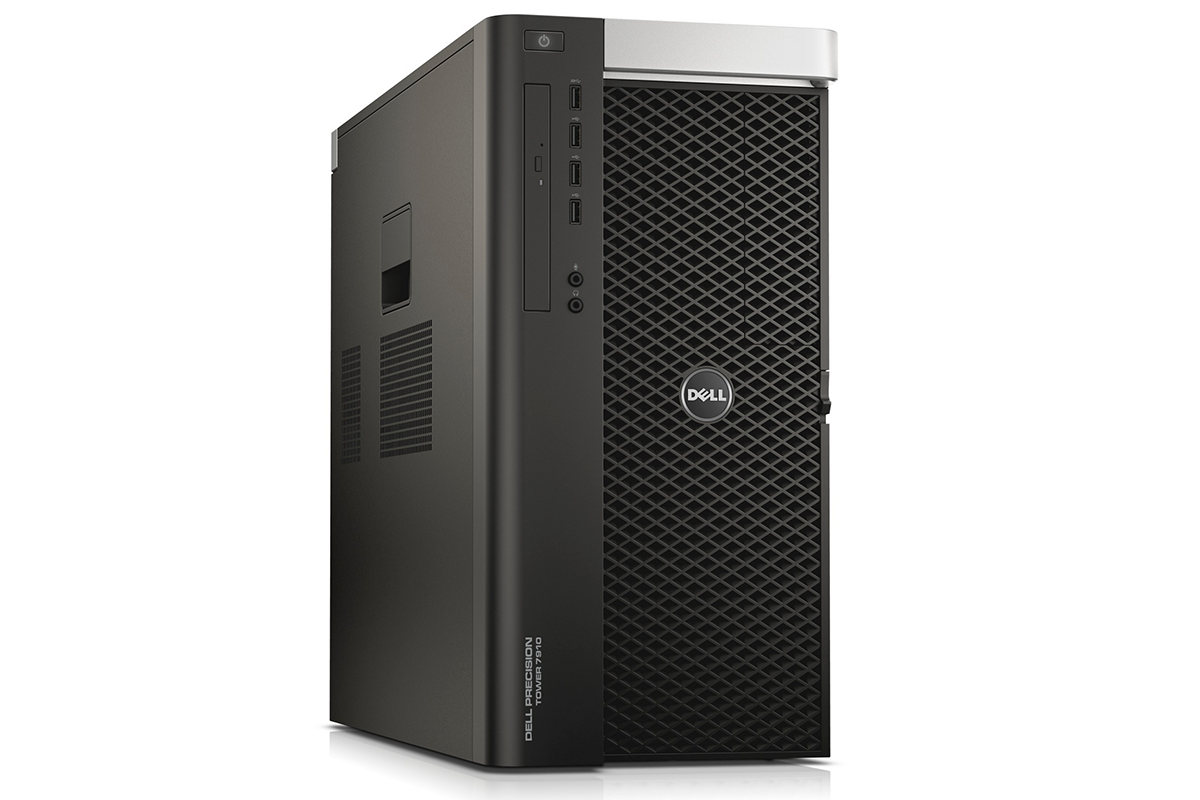
For a further test of the Dell Tower 7910, transient processes were compared with air and liquid cooling at the same air flow rate. To simplify the relationship between the heat capacity potential [cp] and the thermal resistance [R] of the cooler, we will use the thermal time constant:

where tau is defined as the time required to reach 63% of the total temperature rise to steady state. The time constant makes it possible to physically measure the characteristics of heat capacity and thermal resistance of the cooler according to standard methodology.
The figures below show the time response for an air and liquid cooling system with a high TDP CPU. The temperature difference between the two solutions shows the advantage of thermal resistance due to the use of a large surface area of the radiator in the LSS. The delay between them shows the difference in time constants.

Thermal characteristics of liquid cooling compared to air cooling in a T7910 workstation.As you can see, the time it takes for the liquid cooling system to achieve a Tau air temperature is 97 seconds: this is more than 3 times the rate of traditional air cooling (30 seconds). As shown in the figure above, the absolute temperature between air and liquid cooling is reduced by 5 °, indicating a 12 percent increase in performance.
What does all this mean for the user of the Tower 7910 workstation? A liquid cooled system will operate at a much slower fan speed. In addition, as can be seen below, while maintaining the load and constant CPU temperature, the speed of rotation of the processor fan 1 is more than 2000 rpm, while the speed of rotation of the processor fan 2 slightly exceeds 500 rpm.
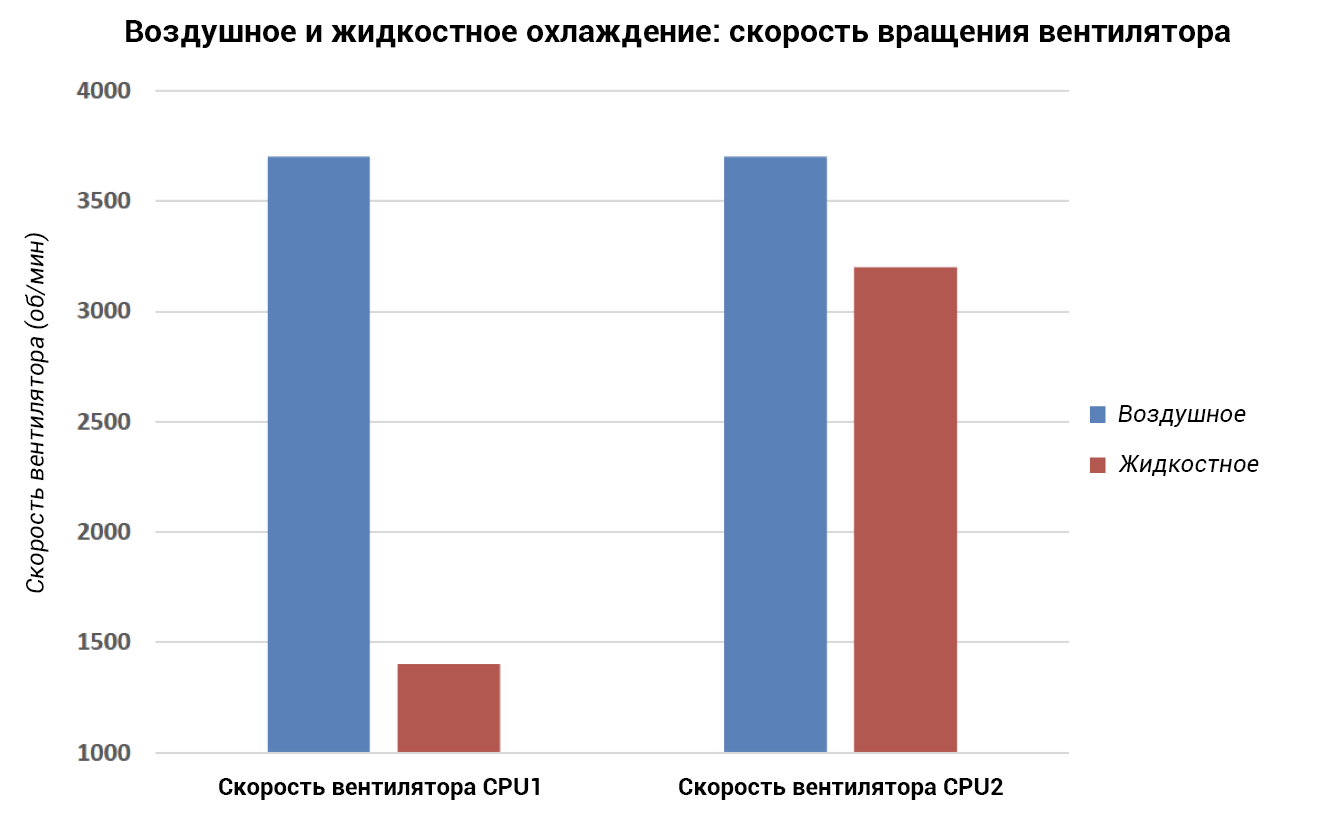
Sound parameters were measured for two scenarios. The first is an extension of the previously presented analysis for TDP, while in the second test example, a scenario typical of CAD users was investigated. The acoustic response of the system was determined at maximum load (160 W of processor power). The figure shows the transient response of the system under this load for liquid and air cooling. As can be seen, between them there is a significant lag in the acoustic response. For reference, the noise level of 30 dBA is equivalent to silence in the library, and 45 dBA - the full conference room.
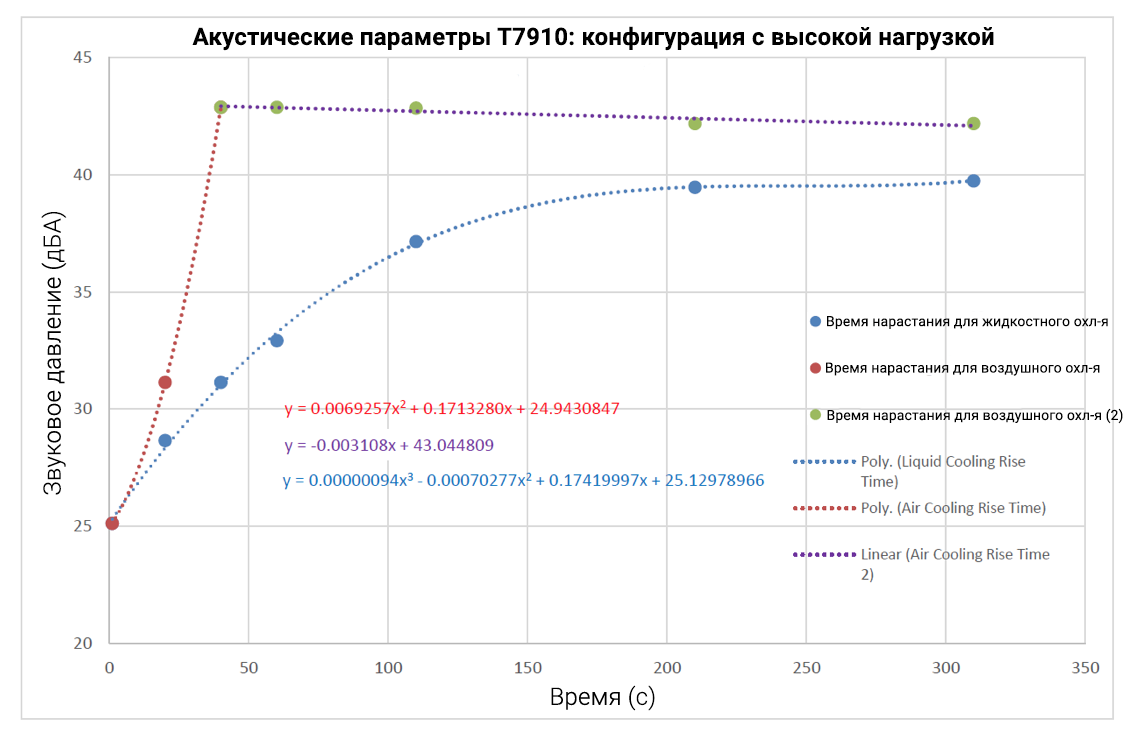
From the test it becomes clear that the total time to a stationary acoustic state for air cooling is 40 seconds, and for a liquid one more than 200 seconds. In addition, by sampling, you can analyze the benefits associated with the use of liquid cooling. The table below illustrates the sampling rate of 25 seconds and the acoustic characteristics at each time point. As can be seen from the data on the 50-second time interval, the acoustic difference between the liquid and air cooling is almost 10 dB, and when approaching the 125-second mark - 5 dB. This result indicates that the heat capacity of the fluid provides an acoustic advantage over time.
Comparison of systems with air and liquid cooling at sampling.The second case under investigation is a test with popular CAD software (3D CAD). For this application, a test script was used that performed general actions: pan, zoom, rotate, create parts, build, etc. The power and CPU load were measured over time. The first figure shows the uneven load of the CPU with respect to time, as well as% of the maximum measured power. This conventional variable load application shows the high potential of a cooling solution with a high heat capacity such as liquid cooling.
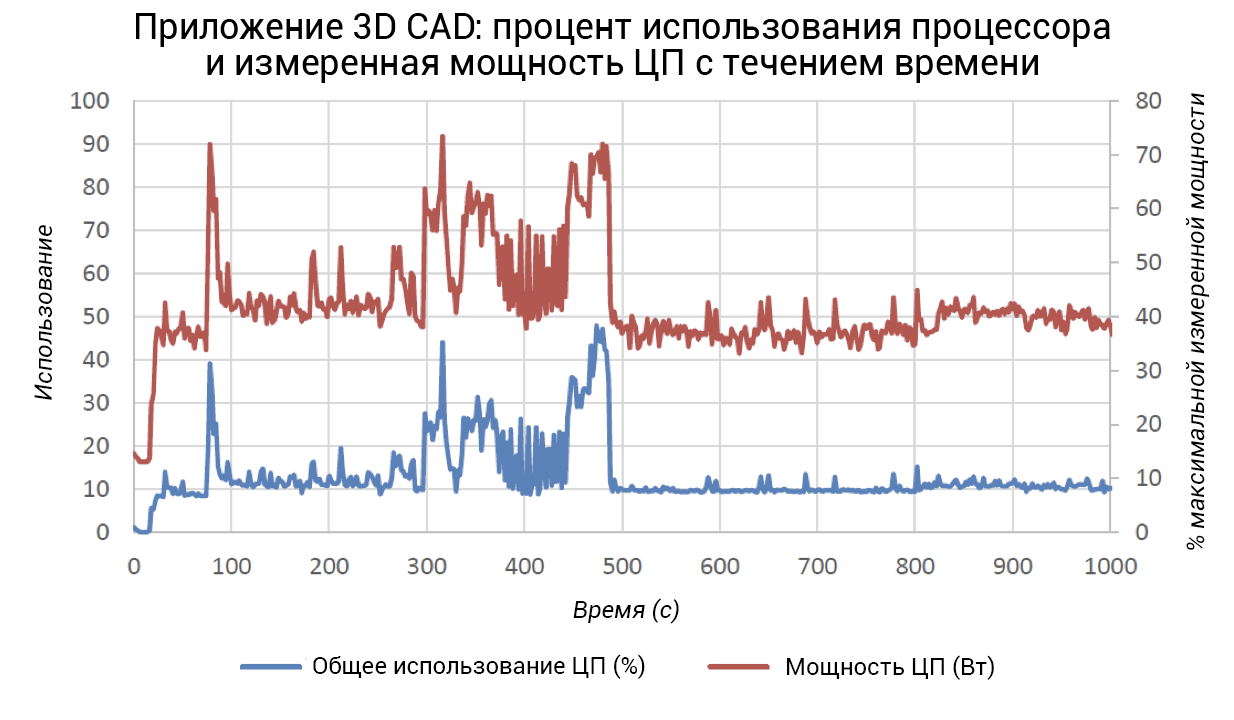

The figure below describes in more detail the time interval from 250 to 500 seconds. In this interval of the test scenario, it can be seen that the load has a local maximum approaching 70% of the maximum measured power. At the same time, local minima are close to 35% of the maximum measured power.
The high heat capacity of liquid cooling should absorb these peaks without fan acceleration as fast as with air cooling. For further analysis of the sound pressure difference between the two systems, acoustic data were determined in discrete time steps.
The figure shows the dependence of sound pressure on the time between air and liquid cooling. The data clearly illustrate the benefits of LSS for a workstation user. Liquid cooling in this case does not exceed the level of noise 28 dBA, and the system with air reaches a peak of more than 40 dBA.
Using the data shown in the figure, the average rate of change for liquid cooling was defined as 0.054 dBA / s, while for an air-cooled system this figure was 0.38 dBA / s. In LSS, the rate of change in the 250-second segment was 86% lower.
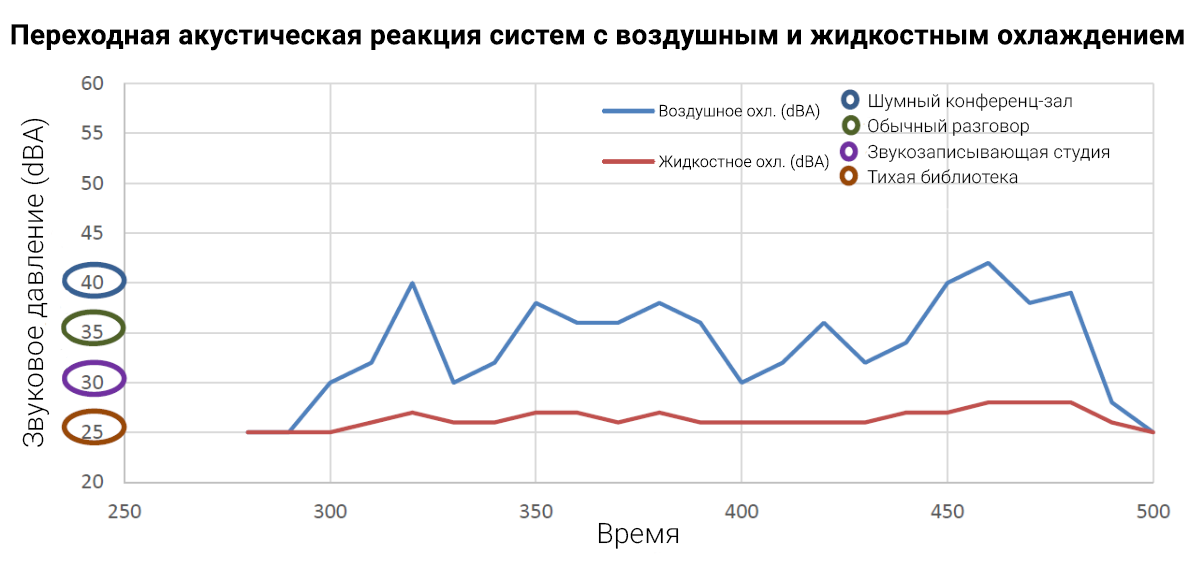
findings
The study demonstrates significant acoustic benefits for customers of Dell Precision workstations with the Dell Precision Tower 7910 liquid cooling solution. These advantages are evident in both heavy thermal loads and in the case of typical interactive operations. For both scenarios, the natural heat capacity of the liquid provided a threefold increase in the time required to achieve the maximum fan speed, compared with the best-in-class air-cooling. And for applications with variable load, the acoustic response in a transient mode gained 10 dB advantage.
Thus,
liquid cooling can make
the Tower 7910 workstation much quieter - both for engineers or traditional CAD users, and for those who work with intensive computing applications (for example, are engaged in modeling and analysis tasks).