Part 2. Very Heavy Fuel
Previous part 1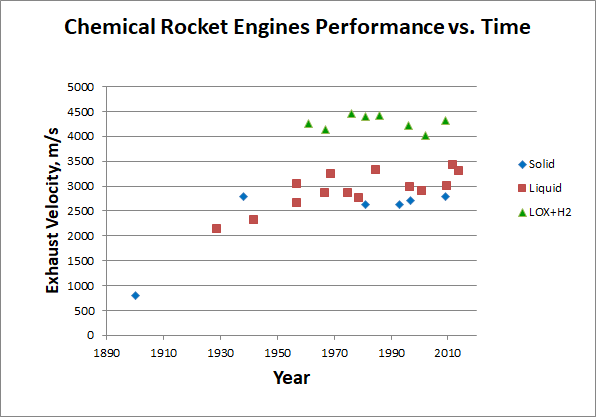
Before you is the International Space Station. A mass of 420 tons and a cost of $
20 billion
[ 20 ] :
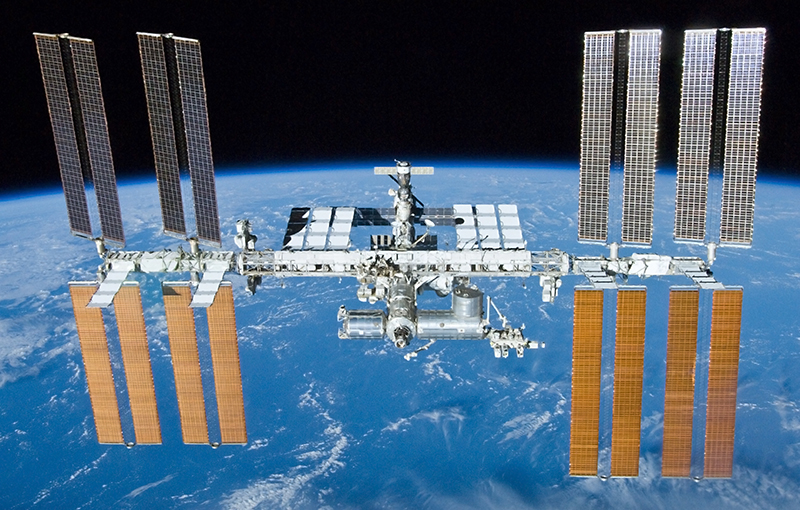
Her kinetic energy, the good old
E =
mv 2/2 , is 1.3 * 10
13 joules. Adding potential energy at an altitude of 400 kilometers, we get 1.4 * 10
13 J.
How much gasoline should I burn to get this energy? It turns out not so much. 350 tons in total. This is approximately
[ 200 ] one-day energy budget of Ulan-Ude.
How is it that far from being the richest city in the world, in one single day, it manages enough energy to accelerate the ISS to orbital speed; however, we have one station for the whole world, and it costs indecently big money?
The answer
lies in the obvious from the anatomy of the launch vehicle.
The carrier, as a minimum, is obliged to include:
- Payload. Otherwise, why is it even needed?
- At least one engine.
- The body, all this connecting.
- And, of course, fuel. As a working body and, in most cases, as a source of energy.
And at the last point, the problem is rooted. To raise and steal a certain minimum of fuel, you need ... right, extra fuel! On the rise of which you also need fuel! And this cheating continues for a long time. Of course, it converges, otherwise we would not fly anywhere at all. But according to the results of convergence, a modern rocket, even a multistage one, even qualitatively designed and executed, consists mainly of this very fuel.
Well, a textbook example, Saturn V is not the newest, but one of the most effective missiles in history
[ 30 ] :

[Original image from NASA History [
40 ]]
Starting weight - 2970 tons. About 2670 of them are fuel. Of which 2160 burn out in less than three minutes of the entire lunar expedition. Given that the kinetic energy of the payload in orbit is "worth" only about 100 tons of fuel.
It turns out that the main difficulty in entering orbit is not a lack of energy. Her earthlings are in abundance enough for the kinetic energy of not only stations, but at least cruise ships in orbit. The problem is different: our fuel is too heavy. Too much of it is needed in kilograms to collect the amount of energy required for flight. Why most of the fuel poured into the rocket goes to transport the same fuel. Actually, the Tsiolkovsky formula, linking the starting
M and the final mass of the rocket
m with the gained speed
V and exhaust exhaust rate
u , tells us the same thing:
M /
m = e
V / u [2]
At first glance, it is not entirely clear what does the energy content per kilogram have to do with it? But it's simple. It "sits" in
u , at the rate of flow. For a chemical fuel, it is limited to (and in the first approximation it is equal to)
u = √ (2
q ), where
q is the specific heat of combustion. Which is the energy content per kilogram. And when this
q "does not reach", the mass ratio at the start and finish turns out to be exponentially huge:
M /
m = e
V / √ (2 q ) [3]
Or
V = Ln (
M /
m ) * √ (
2q ) [3a]
A few notes, for bores and for clarity1. Yes, there are more accurate expressions for the flow rate than
u = √ (
2q ). When I passed the test on them, Gorbachev "gave" the USSR. But these formulas are complicated, scare the readers, and take into account the effects that are not important here.
u = √ (
2q ), although it overestimates the answer by 10-30%, adequately describes the dependency we are interested in. And yes, there is such a thing as a specific impulse, but in this particular article the flow rate is more convenient to use.
2. In principle, nothing forbids even a chemical rocket to get an outflow rate
u higher than √ (2
q ). How? Well, let's say, to burn fuel not in the nozzle, but in the generator, generating electrical energy. Then, with this energy, accelerate the fraction
x of the exhaust (0 <
x ≤ 1) to very high speeds. Suppose a plasma electric jet engine
[ 230 ] . And the remnants of the exhaust stupidly reset at zero speed. For simplicity, we assume that all conversions occur without energy loss, with 100% efficiency. Will such a rocket accelerate to greater speed
V with a fixed mass ratio
M /
m (i.e., will it be more efficient)?
The answer is negative. It is easy to solve the equations describing the motion of such a rocket, and get:
V = Ln (
M /
m ) * √ (
2qx )
Those. its final speed will be only √
x of the recruited “normal” rocket with direct fuel combustion (cf. [3a]). And this speed is still rigidly tied to the value of
q .
3. What if the rocket does not run on fuel, but on a battery? Well, let there be a separate working body of mass
m f on board and a battery with energy
E and mass
m b separately.
The first thing to understand here is that the battery should consist of many small “modules” that are discharged as they are tested. For otherwise, we will carry with us a “dead” load of empty batteries. But if so, then conceptually it is no different from conventional fuel on board with a full supply of energy
E and mass
m f +
m b . And if
q =
E / (
m f +
m b ) of such a system is lower than that of a conventional chemical rocket, then it will fly no better.
5. But what about electric rocket engines
[ 225 ] ? After all, they give flow rates of tens and hundreds of kilometers per second, and successful interplanetary maneuvers were performed on them with very modest
M /
m relationships. How so? The point is that EREs are unlocked systems. The working body (mercury, xenon, etc.) they carry with them. But energy is not. Energy comes from solar panels. If, instead, they carried with them ordinary batteries with an energy content per mass
q , then they would have had no higher efficiency, according to the formula [3a].
Article written for the site https://habr.com . When copying please refer to the source. The author of the article is Evgeny Bobukh .So, the rockets are expensive because their extremely lightweight “dry” construction is forced to withstand extremely heavy loads, mainly fuels (and even quickly “pump” it through the THA). And the load is great because our fuel is too heavy
about . Very little in it is placed joules per kilogram.
Let us now understand the reasons for this restriction.
For which we take a closer look at the reaction of hydrogen burning in fluorine, as one of the simplest. In it, hydrogen-hydrogen and fluoride-fluorine pairs exchange partners, creating two hydrogen-fluorine pairs:
H 2 +
F 2 = 2
HFWhere does the released energy come from?
A hydrogen molecule has two atoms. The atoms have electrons. They are “smeared” around the atom in the form of such a cloud, and are connected with the nucleus mainly by electrostatic attraction. Electrons are external, valent, and (except hydrogen) internal, not participating in chemical reactions. After the reaction, hydrogen and fluorine are swapped. The electric clouds of valence electrons are redistributed and slightly change their shape. Something like this:

[Image credit [
295 ]]
The potential binding energy of electrons with atoms in the new clouds is different. In this case (this energy is negative), it is now less than that of
H 2 and
F 2 separately. Where did the difference go? In the kinetic energy of a molecule, the vibrations of its atoms, electromagnetic radiation. All this eventually turned into heat. Which expanded gas and gave cravings.
And here is the crucial moment. Only external, valence electrons are involved in chemical reactions. The density distribution of other electrons (as well as the intensity of the electric field "in depth" of the atom) remain almost unchanged. In chemical reactions, atoms interact with each other as if through "intermediaries", which are the valence electrons:

Now, attention, the question is: what is the maximum possible energy that can be released during such a "permutation"? Obviously, it cannot exceed the sum of the binding energies of external electrons with atoms (in the final and initial products). But these binding energies are well known to us
[ 285 ] . Calculated per atom, they are 1.5–25
electron volts (
eV ) and are expressed in fractions of the Rydberg constant — the fundamental value constructed from the base constants of our Universe:
Ry (in the Gaussian system) =
m e e 4/2 ħ 2 =
1 3 .6 eV [
300 ]
At the same time, both 25 and 13.6 eV are unattainable. For in typical reactions, not all of the binding energy is released, but only its difference between the two configurations, and therefore the practical energy release limit of chemistry is 3-4 eV per atom. In terms of a typical kilogram of fuel + oxidizer, this is equivalent to 20-30 MJ of energy released. This is the value that sets the theoretical maximum gas flow rate of a chemical jet engine with direct combustion
u = √ (2
q ) = √ (2 * 2.5 * 10
7 ) ≈ 7000 m / s. Unachievable, of course, because it does not take into account losses to the internal degrees of freedom of molecules, dissociation, radiation, non-directional thermal motion, etc.
It may seem that the table heat of combustion
[ 240 ] (say, 120 MJ / kg for hydrogen) contradicts the above figure. But the fact is that these heats are usually indicated per kilogram of
fuel , without taking into account the oxidant required for its combustion. The rocket carries both components with it, and if you recalculate the energy released per kilogram of the
mixture (with balanced combustion), a completely different picture emerges
[ 240 ] [ 250 ] [ 260 ] :
Fuel + Oxidizer | Reaction | Heat value per kg. fuel, MJ / kg | For 1 kg of fuel you need an oxidizing agent, kg. | The heat of combustion of the mixture, MJ / kg | The yield on the atom mixture, eV |
Hydrogen + Oxygen | 2H 2 + O 2 = 2H 2 O | 120 | eight | 13.3 | 0.83 |
Kerosene + oxygen | 2C 12 H 26 + 37O 2 = 24CO 2 + 26H 2 O | 43 | 3.5 | 9.6 | 1.02 |
Coal + Oxygen | C + O 2 = CO 2 | 33 | 2.7 | 9.0 | 1.38 |
Lithium + oxygen | 4Li + O 2 = 2Li 2 O | 43.5 | 1.2 | 20.2 | 2.10 |
Boron + oxygen | 2B + 1.5O 2 = B 2 O 3 | 57.2 | 2.2 | 17.8 | 2.58 |
Magnesium + oxygen | 2Mg + O 2 = 2MgO | 25.1 | 0.7 | 15.1 | 3.18 |
Beryllium + oxygen | 2Be + O 2 = 2BeO | 66.6 | 1.8 | 24.0 | 3.12 |
Lithium + fluorine | 2Li + F 2 = 2LiF | 88.8 | 2.7 | 23.7 | 3.21 |
Beryllium + fluorine | Be + F 2 = BeF 2 | 114 | 4.2 | 21.9 | 3.57 |
Dicyano Acetylene + Ozone | C 4 N 2 + (4/3) O 3 = 4CO + N 2 | 16.2 | 0.8 | 8.8 | 1.28 |
As we see, even the most energy-intensive, albeit unsuitable for practical use, fuels provide only 24 MJ / kg of heat during combustion. And we have rested against this limit almost from the beginning of astronautics, which shows a graph of the speed of the expiration of chemical engines, depending on the year of their creation:
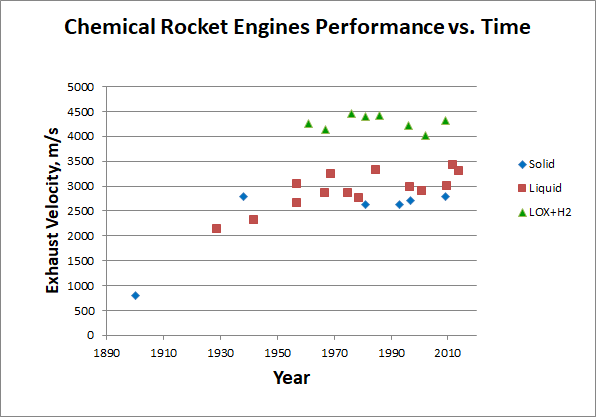
[Early engines according to [
310 ], [
320 ], [
330 ], later - Wikipedia one by one. Data collection
here ]
It seems that the potential of chemical fuels has long been developed. Do not start to store energy in another form?
Continued.References and literature