Part 2. Walk
→
First partFirst, thank you so much for so many good and meaningful comments. Only a lack of time does not allow to answer all of them in detail. But I appreciate both the additions and the links to unread fiction (how much of it!) And corrections. Thank!
There will not be much depth in this section. And there will be a small tour of the outskirts of the pT-diagram, rarely visited even by popularizers of science. What for? To show that the wealth of worlds and phenomena most likely does not decrease with distance from the “
world center of conditions” we are used to. And that, probably, in those places something interesting and plot-generating can occur ... provided that at least one professional writer can think this through with high quality. But this is a separate topic for discussion in the third part. Here we will have just distant worlds. Not in parsecs far, but therefore no less difficult to reach.
Jupiter
This is how it looks outside:

And so, according to modern concepts
[ 490 ] , from the inside:

[Image Credit: Sean Wahl
et. al., [
490 ]]
The scattering of crooked images in the picture means that we still do not know whether Jupiter has a compact core, or whether it is dissolved in supercompressed hydrogen. Therefore, I will confine myself to less serious depths. Thousands of people are about 15 kilometers away. The pressure there is ~ 2 million atmospheres, and the temperature is 6-7 thousand degrees. Hydrogen (which is ~ 90% in Jupiter) is compressed there to a density of 200 kg / m
3 , partially converted into metal and, by its consistency, resembles a cross between gasoline and mercury - if they could dissolve in each other.
But helium in this mixture does not want to dissolve. Therefore, it is collected in drops and sown down with a kind of helium “rain”. In quotes, for it looks more like settling a scrambled mixture of water and oil. And this “rain” does not get anywhere, because the solubility of helium in hydrogen is even deeper and the drops, having disappeared from tens of thousands of kilometers, dissolve completely. All this at 6000 degrees.
Somewhere there or just above the magnetic field of Jupiter is born. In fact, we vaguely imagine the principle of the magnetic dynamo, even the earthly one. It is clear only that the already existing magnetic field somehow cunningly “bends” the convective streams of conducting material in the planet in order to use their energy and thereby self-reinforce. In this, it is similar to terrestrial life, which also “rolls” on energy streams that are available for saddling, be it sunlight or hydrogen sulphide from the depths.
It is possible that the zonal winds of Jupiter, in the form of such cylindrical columns, extend at least to these depths.
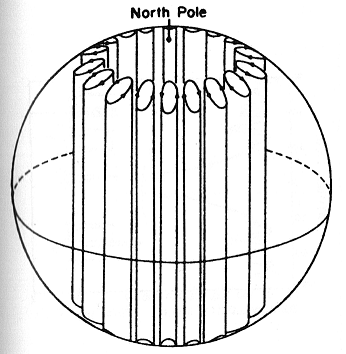
[Image credit [200]]
Suppose we want to learn more about these areas. What are the opportunities?
First thought: electromagnetic waves.
Alas, optics and near infrared allow Jupiter to probe only up to about 4 atmospheres of depth
[200] - that is, some kind of kilometers for 40. At millimeter waves, you can “break through” up to 100 atmospheres
[200] , these are kilometers 260. Juno, " Listening "on a wave of 50 cm can hardly disassemble something up to ~ 550-600 kilometers, where the pressure reaches 1000 atmospheres
[ 420 ] , and the temperature - 1300 Kelvin. But in the scale of the image from the header, these are only nine pixels:

We also need ten times deeper - about under the edge.
Maybe send a descent vehicle?
Galileo in 1995 was able to plunge for 160 kilometers, up to 22 atmospheres and 152 degrees Celsius. Two pixels.
The limiting depths of the future probes of Jupiter, still being considered seriously
[ 460 ], are 200 atmospheres. Five points, or 330-340 kilometers.
And if you really dream on a grand scale, then you can come up with a scheme. We take a spherical bathyscaphe of single-crystal diamond. With walls a couple of meters thick, reinforced with rhenium (I don’t think that will help, but how it sounds!) We cover with something waterproof. We put on it a nuclear reactor for active cooling. We fill the instruments and dumped on the planet. Communication - small pop-up probes. This, of course, is fiction - but fiction is still scientific.
Diamond, the strongest substance in compression, withstands
[ 410 ] a pressure difference of about 100 GPa, or a million atmospheres. Up to the geometry of manufacturing such a probe will be able to look into Jupiter for 8-9 thousand kilometers. This is close to helium rain and magnetic dynamo. But even this is only 1/8 of the radius of the planet ...
What can be observed in the process of such a dive?
First, we will break through clouds of ammonia NH
3 (0.7 atm), ammonium hydrosulfide NH
4 SH (2 atm) and water H
2 O (7 atm).
Atmospheres on a hundred will become completely dark.
At ~ 500 kilometers, with 500 atmospheres and a temperature of 1064 K, the probe will pass through a thin haze of clouds of ... gold. At least, if you believe the work
[ 440 ] . Like it or not, no one, of course, knows. But gold is rather inert and, for metal, relatively volatile, so it does not contradict physics.
At a thousand atmospheres, the gas density will reach ~ 20 kg / m
3 - and it will not be possible to simply consider this medium as a gas. Approximately in the same place can be located clouds of sodium sulfide Na
2 S.
At 700 kilometers, at 4800 atmospheres and 2000 Kelvin,
[ 440 ] clouds of magnesium silicate MgSiO
3 are floating outside. However, it is difficult to notice this in appearance outside the window, and in general it is unlikely that he will impress anyone:

Because it is simply the glow of a substance heated to 2000 Kelvin. Not only different from the heat of a glass melting furnace. And this picture looks far into the distance a few decimeters. For approximately this
[ 430 ] is the transparency of matter in those conditions. What it illustrates: not only our phatasia, but also our ways of perception are helpless in conditions that are too far from “normal”.
But our theories continue to work in them and predict a lot of interesting things.
At half a million atmospheres (5600 kilometers, 5100 degrees), hydrogen begins to dissociate, which can be considered the beginning of its transformation into metal. The closest visual analogy of this is the dissolution of sodium in liquid ammonia
[ 830 ] . As the concentration of free electrons increases, the solution darkens, loses transparency, and increases electrical conductivity.
Below 2 million atmospheres, iron turns out to be soluble in metallic hydrogen
[ 450 ] , and with stony minerals it occurs above 5 million atmospheres and 10 thousand degrees
[ 450 ] . What is achieved on a quarter of the "depth of Jupiter". Further, its matter is probably a sort of superdense, overheated, partially ionized “broth” made of metallic hydrogen with admixtures of other elements.
Can there be something more intriguing, rather than evenly mixing this “broth”? This question does not give much rest.
Thus, according to [
730 ], the deep bowels of Jupiter may have a multi-layered structure. With jumps in the concentration of impurities between the layers. Well, it seems like we have on Earth, when cold, smoke-filled air is gathering a clear “cake” of smog over the city. Only on Jupiter are these jumps due to the difference not in temperature, but in chemical compositions. For example, more SiO
2 is dissolved in the bottom layer - and this makes it heavy enough to resist convection. It is clear that on the boundaries of such layers there will be sharp drops in both temperature and chemical composition. For example, at a very large depth, substance AB decomposes into components A + B. Above, they are ready to connect back, but this process is slow. The absence of convection prevents them from rising and mixing with the atmosphere. As a result, right under the boundary of the layer, an excess of separated A and / or B. If there also appears
something capable of catalyzing the reaction A + B -> AB, then it will have a source of chemical energy to evolve into
someone . Of course, for the emergence of this life is very small - but enough so as not to reject the very idea with a run-up.
Could this conditionally imaginary life somehow contact us? Climbing up it is definitely contraindicated. Habitual to pressures, even at times compressing a stone, to a medium where iron is a gas and a solution component, in the upper layers of Jupiter, it will simply evaporate and disintegrate like foam in the wind.
Electromagnetic waves, as we have already established, from such depths do not pass. At least because of the layer of metallic hydrogen.
Gravity? Density structures in the depths of Jupiter are well captured. If they are tens of thousands of kilometers in size.
A magnetic field? Juno magnetometers "see" to the level of metallic hydrogen, i.e. two million atmospheres. There may be hope for them.
Modulation of the neutrino flux? We have so far from the whole of the Sun, these particles are difficult to register.
And there remains ... sound. Ordinary sound waves. Which, as shown in [
500 ], are able to pass tens of thousands of kilometers in Jupiter, and which we have learned how to detect recently. True, we are talking only about frequencies of about one
milli hertz. On such fluctuations, the transfer of the text you read will take about 300 years. Having encoded it with a Morse code, we will be able to quickly transmit it by radio to a nearby star. Sometimes the dissimilarity of conditions can be a far greater barrier than physical distances.
[Disclaimer. In order to show the whole picture, I have mixed materials from [200,
420 ,
430 ,
440 ,
450 , 470,
480 ,
490 ,
500 ,
730 ] in this note. They are often based on incompatible models, data, and assumptions about the composition of the planet and the behavior of matter. There are absolutely no radical contradictions between them, but it is worth remembering that putting them together is an applied Frankenstein breeding. Allowed for viewing purposes, but only.]
The moon in the tanker
The school teaches that the moon has no atmosphere. This is not entirely true. Somehow, the moon still has some sort of gas envelope. True, it is about 15 times shorter than ours. Therefore, if the entire lunar “air” is squeezed to earthly conditions, then it will be enough except for filling a decent gym, and you can take away all this atmosphere on a single truck with a solid tank.
However, in this rarefied area on the pT-diagram, something interesting happens that many people annually publish a bunch of articles, come down to discuss them, and even launch an interplanetary station (LADEE) specifically to study the lunar atmosphere.
One such work
[ 720 ] based on materials from LADEE is devoted to lunar argon. From it, basically, the local atmosphere and consists. Only here "consists" and "atmosphere" need clarification. Because for some reason, argon over some parts of the Moon is several times larger than over others, and from the time of day its amount changes altogether tenfold. In essence, this is not a “gas envelope”, but a kind of cloud that breathes, changes size, wanders, is sensitive to temperature, ionization by the solar wind, soil composition, and is able to temporarily “stick” to its surface or permanently settle in “cold traps” at the poles . Lost in the end into space and fueled by the decay of potassium in the lunar crust. The alleged source of which may be responsible for the discovered “argon hump” over the western lunar seas.
[ Article written for the site https://geektimes.ru/ . When copying please refer to the original. The author of the article is Evgeny Bobukh. You can support the author by cryptocurrency at the addresses indicated in the profile . ]Another publication
[ 540 ] explores lunar ... atmospheric radon. He was caught there literally by atoms, but was able to build a map of the distribution of lunar polonium in a certain area:
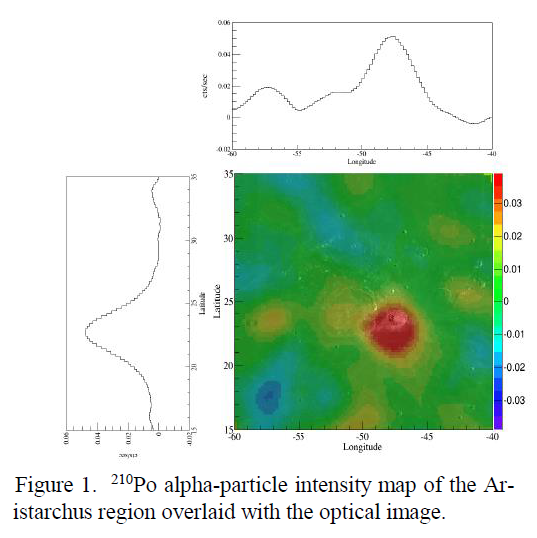
This result is displayed visually, but the best analogy should be the sense of smell. When the station was in orbit, turn by turn, it was exactly what snatched the radon atom atoms, and built a picture of the world from them. To the human eye, the lunar atmosphere does not appear decidedly anything interesting:
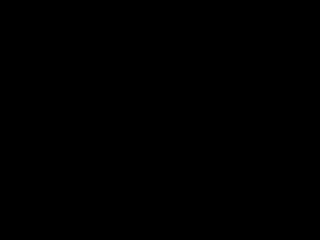
Too sparse, and everything that happens in it - all these plasma interactions, sorption of particles, movement along the lines of local magnetic fields - have to be drawn with schematics and formulas.
While lying. There is one mystery half a century ago with quite a visual representation.
Back in 1968, the landing station of Surveyor 7 photographed
[ 550 ] [ 555 ] from the surface of the moon something resembling ... a dawn:

[Image credit: NASA, [
555 ]]
American astronauts in 1972 with the lunar orbit also observed
[ 560 ] similar phenomena:
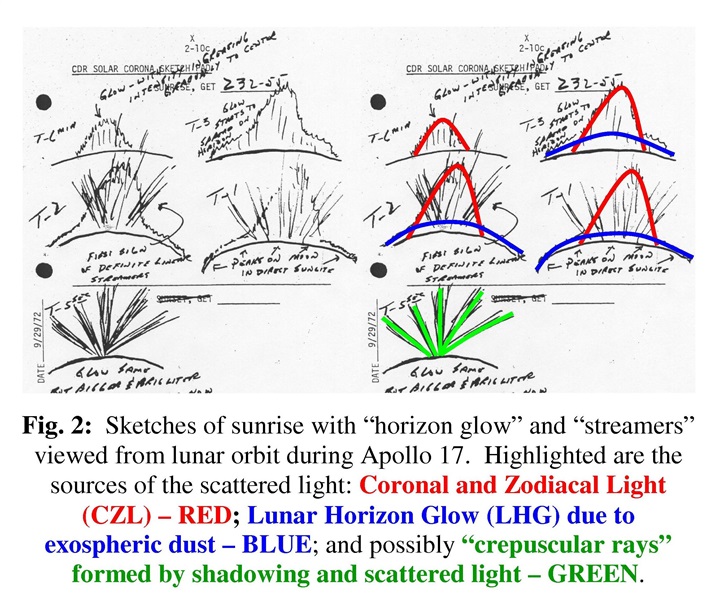
[Image credit: NASA, [
560 ]]
However, what can shine there in the rays of the Sun if there is no air? Today it is considered that it is ... dust. Microscopic electrically charged particles hovering literally in meters above the surface, create a "dawn" of lunar "mornings".
All is good, but what raises them? Electric field between the areas shaded and open to the solar wind
[ 740 ] ? Microscopic fluctuations of electric charge
[ 750 ] ? Microprobe with “explosion” of regolith particles
[ 760 ] ? The attacks of micrometeorites
[ 770 ] (although it is unlikely - I conjecture from myself). Why do some modern studies
[ 780 ] of these lunar dawns fail to point out? Do these particles fly up as "fountains" as the terminator moves, do they float in a uniform layer? Can form a so-called plasma crystal, the structure from our point of view is very unstable - but quite orderly?
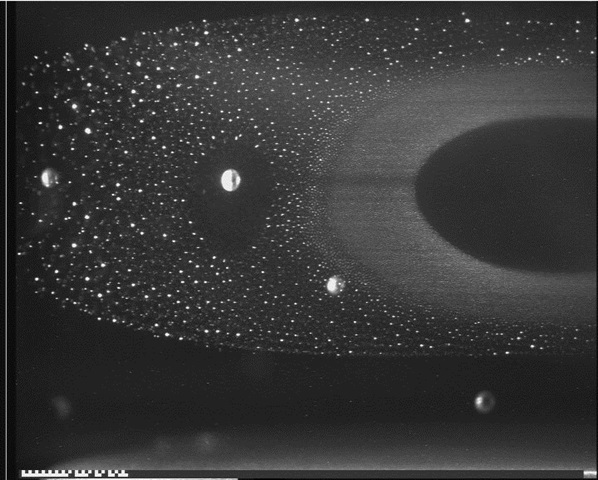
[Plasma Crystal Experiment at the International Space Station. Image credit: phys.org, [
790 ]]
The question, however. Pretty much open.
On a daily basis, the study of these rarefied matters is not worth the effort. Some gases and impurities fly in almost complete vacuum in microgram quantities per hectare. Dun - and everything will disappear. By the way, and disappeared. Each landing of the Apollo on the moon diluted the lunar atmosphere with engine exhaust about twice.
But just as fragile and ephemeral our earthly matter may seem from the point of view of (purely hypothetical) inhabitants of neutron stars. Does this mean that in our matter there is no structure, complexity and nothing worthy of study?
Neutron stars
We have much more questions about them than answers, so almost everything in this section is only more or less substantiated hypotheses, drawn mainly from the review [40]. Good, by the way, and in Russian.
So, neutron stars, they are pulsars - they are objects with a mass of the Sun, but the size of a city (20-30 km), which causes gravity on them reaches ~ 10
1 1 g. With temperatures of millions of degrees, pressures and other parameters, also crawling out of the edges of the screen. I think they look something like this. If you look through a very, very dark filter and do not burn due to radiation:

[Based on Image by NASA. Background such as this primary's brightness]
Neutron stars have, first, nuclei, about which little is known, apart from estimating the pressure in the center: ~ 10
29 atmospheres. Theorists do not even know what their matter consists of. But its density probably greatly exceeds the density of even the atomic nucleus (2.8 * 10
14 g / cm
3 ). A piece of such matter the size of a bacterium creates on its surface the same force of gravity as the Earth does on its own.
Secondly, neutron stars have something like a mantle and crust. I quote
[40] : “
The substance of the deepest shells adjacent to the nucleus of a neutron star is a neutron liquid into which atomic nuclei and electrons are immersed. The neutrons and electrons in these layers are strongly degenerate, and the nuclei are neutron-rich — the number of neutrons in them can exceed the number of protons by several times, and only a huge pressure keeps them from decaying. The electrostatic interaction of the nuclei is so strong that it arranges the nuclei into a crystal lattice that forms a solid star crust. The mantle may be located by the core and the core of the star (though its existence is not predicted by all modern models of dense nuclear matter.) The atomic nuclei in it take on exotic forms of extended cylinders or planes <...> Such a substance behaves like liquid crystals <... > The neutron star's crust is divided into internal and external. The outer crust is distinguished by the absence of free neutrons. The boundary lies at a critical density <...>, above which begins the "leakage of neutrons" <...> from the nuclei. <...> With decreasing concentration The electrostatic interaction between the ions weakens, and as a result, instead of the crystal lattice, the Coulomb fluid acquires thermodynamic stability. The position of the melting boundary, which can be called the bottom of the ocean of a neutron star, depends on the temperature and chemical composition of the shell. "
Chemically, it is most likely iron. But, do not forget, compressed to a density of 10
5 - 10
9 g / cm
3 , compared with which our steel is a vacuum in a fluorescent lamp!
Neutron stars also have atmospheres. From plasma of hydrogen, helium, carbon and iron, under a million degrees preheated. But the thickness is only in millimeters. And layers of different composition and density are squeezed into these millimeters (Flat world! Right word, that's what the novel would write! But who has enough imagination?) These atmospheres are usually opaque and glow; as a rule, it is their radiation that we see, looking at a neutron star through a telescope.
Finally, neutron stars have a magnetic field. The typical intensity of which is ~ 10
12 times that of the earth. The pressure of such a field is ~ 10
1 6 atmospheres. This is enough to flatten the atoms, forcing their electron shells to stretch along the field. And to bring to life chemical bonds, unthinkable on Earth:
"
A strong magnetic field makes the He 2 molecule and its ions He 2 + , He 2 2+ , and He 2 3+ stable, which do not exist outside the magnetic field." Although
, “at densities, temperatures, and magnetic fields characteristic of neutron stars, the content of such molecular ions is extremely small ... ” But further on page 818: “
Ruderman [512] suggested that a strong magnetic field can stabilize polymer chains stretched along magnetic field lines, and that the attraction of these chains to each other due to the dipole-dipole interaction can lead to the formation of a condensed state. only of chemical elements easier oxygen and their condensed phase polymerization takes place either in ultrahigh field or at a relatively low temperature ... ". Further, "<
...> Medin and Lai <...> in [359] calculated the equilibrium density of saturated steam for the atoms and polymer chains of helium, carbon and iron over the corresponding condensed surfaces <...> " and showed that their existence is compatible with conditions near the surfaces of neutron stars. Similar assumptions, by the way, were expressed
[600] by Dong Lai (Dong Lai) with respect to the chemistry of the atmospheres of white dwarfs.
That is, yes, the new chemistry. "Polymers of helium in a strong magnetic field."
And where there are polymers, there you can present the storage of information at the molecular level. Only, what kind of science fiction can imagine and qualitatively describe it? No, put into action "neutronoids" - just spit. But who can make them convincing? Who can, starting with physics, build all the chemistry, biology, society, psychology and intrigue? Who, finally, will be able to step over the abyss of the world view between a creature living in a degenerate neutron fluid and us?Right.
That's because, probably, (almost?) And there are no such works. Read Landau, he is much more entangled and convincing.However, something useful from this mental experiment can still be extracted. Namely, to introduce a classification of civilizations according to the stability of their constituent matter to the interstellar medium.Class 1. Stable. They are well experienced conditions of interstellar travel. Imaginary example: some thinking stones.Class 2. His carriers do not tolerate a vacuum. But they have a substance at hand that can be used to make a spacecraft. This is us.
Metal, glass and ceramics are stable in space.Class 3. Locked. And they, and all their matter falls apart outside the usual conditions. They even do a spacesuit from which. The hypothetical inhabitants of the depths of Jupiter or neutron stars fall into this class. All the substance available to them, beyond the colossal pressures, will simply transfer to another state of aggregation.Therefore, if I were a resident of a neutron star, I would still think about modulating the radio signal of a pulsar in order to communicate with its own kind. If you don’t fly, then you can’t leave such a powerful (albeit narrowly focused) source without use. After all, this is their only chance to overcome the interstellar distances, at least informationally.A billion years after the end of the world
A typical stone planet is 1-6% by weight of calcium [15] . For definiteness, we assume that 3%.0.187% of natural calcium is [ 610 ] is the Ca-48 isotope, which has a weak natural radioactivity. His half-life is enormous: 6 * 10 19 years. Each decay produces 4.27 MeV of energy, of which about 3 MeV falls on positrons [ 620 ] , and therefore, goes into heat.Based on these data, we calculate that a cubic meter of a typical stone planet emits 7 * 10 -16 watts of heat from calcium decay. In comparison with the flow of energy from the Sun or natural radioactivity, the figure is, of course, negligible.But neither the sun nor the uranium is eternal.Imagine: in the courtyard of the 1st of January 3 * 10 of the 19th year of our era. Uranium, thorium, and potassium have long disintegrated and do not exist in nature. Innumerable epochs burned out all the stars. Relic radiation cooled to nanokelvinov. It is not clear, however, how fast white dwarfs cool down; but in any case, by ~ 10-15- year they are not hotter than 5 K [ 530 ] and scattered from each other further than modern galaxies. The universe is empty, cold, dark, without form.But the planets, separated from each other by terrible distances, the planets continue to quietly warm and shine. Due to the ongoing breakdown of calcium.It is easy to calculate that thanks to this energy source, a body the size of the Earth will be able to maintain the surface temperature at ~ 0.4 K. Consider that at ~ 1 K the thermal conductivity of stony materials drops to 10 -2 - 10 -3 W / m 2 * K [520] . Which means, how again it is easy to calculate that the bowels of such a planet can be heated to 1-5 degrees of heat!You ask - what interesting things can happen in such a warm cold? I do not know.
But I know that these phenomena have in stock ~ 10 20 years. Time, incomparable with anything familiar, because in today's universe, and the second, then so much will not be typed. What events are too slow to even consider them today as processes become dominant at such times s s scale?After all, no one has canceled diffusion in a solid body, including quantum one, and diffusion with a reaction capable of creating self-ordered structures [ 510 ] . If the transport of terrestrial microscopic substances is based on diffusion in a liquid, is it possible to imagine the same thing in a solid body, only 10 11 times slower?No one has canceled the transition of metals to superconductivity, with the subsequent circulation of the currents trapped by them.Finally, no one has canceled helium. Which at the specified temperatures can liquefy, go into a superfluid state, leak through the pores and cracks in the stones, freeze and thaw again, shrink and expand, thus ensuring the transfer of matter on a global scale.You ask, where does helium come from? So from bismuth! The land of one billionth mass consists of it. And bismuth consists entirely of the alpha-active isotope Bi-209 with a half-life of 1.9 × 10 19 years. And alpha particles are helium. By 3 x 10 19 -th year, used on most of the bismuth disintegrate, selecting about 10 14kilograms of helium, which is enough for a modest atmosphere. Hold it at such temperatures is not something that the Earth, any Ceres can.My human imagination excitedly tossed, sensing the unusual possibilities opened up by such a breakthrough unoccupied time ... and succumbs. It passes and is lost without groping for either physical or household intuition on such a scale.Let us close this curtain, leaving the future to the future, and return to more everyday issues.To the third part.Literature and links15. Katharina Lodders and Bruce Fegley, Jr. The Planetary Scientist Companion. New York, Oxford, Oxford University Press, 1998.
40. А. Ю. Потехин. Атмосферы и излучающие поверхности нейтронных звёзд. Успехи физических наук, август 2014, том 184, №8, DOI: 10.3367/UFNr.0184.201408a.0793, стр 793-832.Очень советую прочитать хотя бы первые главы.
200. Patrick Irwin, Giant Planets of our Solar System, An Introduction, published by Springer in association with Praxis Publishing, Chichester, UK, 2006, ISBN 3-540-31317-6
410.
https://en.wikipedia.org/wiki/Material_properties_of_diamond420. Juno microwave radiometer:
http://www.irmmw-thz2014.org/sites/default/files/F2_D-39.1_Janssen.pdf (Juno at Jupiter: The Juno Microwave Radiometer (MWR), Michael A. Janssen, Shannon T. Brown, John E. Oswald, and Amarit Kitiyakara, Jet Propulsion Laboratory, California Institute of Technology, Pasadena, CA, 91108 USA)
430. The Interior of Jupiter,
https://authors.library.caltech.edu/39188/1/Stevenson_2004p35.pdf , Tristan Guillot, David J. Stevenson, William B. Hubbard, Didier Saumon
440.
http://solarsystem.wustl.edu/wp-content/uploads/reprints/1994/No49%20Fegley&Lodders%201994%20Icarus.pdf , Chemical Models of the Deep Atmospheres of Jupiter and Saturn, Bruce Fegley, Jr., and Katharina Lodders, ICARUS 110, 117-154 (1994). Да, работе скоро четверть века и новых данных с тех пор километр. Но авторы — умные и известные люди и вряд ли совсем уж грубо ошибались, так что хотя бы в качестве иллюстративного материала эта работа должна быть вполне пригодна. К тому же, мне почему-то не попадалось более свежих исследований на эту тему…
450. Giant Planets, Tristan Guillot, Daniel Gautier, Treatise on Geophysics, 2nd Edition 00 (2014) 1–42,
https://arxiv.org/abs/1405.3752460. Overview of Mission Architecture Options for Jupiter Deep Entry Probes, Presented by Dr. Tibor S. Balint at the Outer Planets Advisory Group Meeting, Boulderado Hotel in Boulder, Colorado, June 910, 2005,
https://www.lpi.usra.edu/opag/meetings/jun2005/presentations/JDEP_OPAG_presentation.pdf470. Structures of the Planets Jupiter and Saturn, A Kerley Technical Services Research Report, Gerald I. Kerley, December 2004. Несколько примитивная и устаревшая модель, но даёт оценку параметров в центре ядра Юпитера и соотношения давление-плотность и давление-радиус. Пусть не совсем точно, но для увязки данных полезно.
480. A Preliminary Jupiter Model, WB Hubbard, and B. Militzer,
https://arxiv.org/pdf/1602.05143.pdf490. Comparing Jupiter interior structure models to Juno gravity measurements and the role of a dilute core, SM Wahl, WB Hubbard, B. Militzer, T. Guillot, Y. Miguel, N. Movshovitz, Y. Kaspi, R. Helled, D. Reese, E. Galanti, S. Levin, JE Connerney, SJ Bolton. Confidential manuscript submitted to Geophysical Research Letters, Jul 2017,
https://arxiv.org/abs/1707.01997500. Seismology of Giant Planets, Chapter 14 of the book Extraterrestrial Seismology — Cambridge University Press (2015), Submitted on Arxiv on November 6th, 2014, Patrick Gaulme, Benoît Mosser, François-Xavier Schmider, Tristan Guillot,
https://arxiv.org/abs/1411.1740?context=astro-ph.EP .
510. Reaction-diffusion:
https://en.wikipedia.org/wiki/Reaction%E2%80%93diffusion_system520. A low temperature thermal conductivity database, Adam L. Woodcraft, and Adam Gray,
http://reference.lowtemp.org/Woodcraft_LTD13_materials.pdf530.
https://en.wikipedia.org/wiki/Black_dwarf#Formation540. RADON GAS EMANATION ON THE LUNAR SURFACE OBSERVED BY KAGUYA/ARD. K. Kinoshita, K. Kojima, M. Itoh, T. Takashima, T. Mitani, K. Yoshida, S. Okuno, and J. Nishimura, LPSC 2016,
https://www.hou.usra.edu/meetings/lpsc2016/pdf/3070.pdf550. Surveyor Observations of Lunar Horizon-Glow*, JJ Rennilson and DR Criswell, 13 August 1973. The Moon 10 (1974) 121-142. © Kluwer Academic Publishers,
http://articles.adsabs.harvard.edu/full/1974Moon...10..121R555.
https://www.nasa.gov/topics/solarsystem/features/leaping-lunar-dust.html560. Зарисовка лунных «зорь» астронавтами:
https://www.nasa.gov/ames/ladee-project-scientist-update/600. Dong Lai, про магнитную химию в белых карликах:
http://www.nature.com/news/stars-draw-atoms-closer-together-1.11045610.
https://en.wikipedia.org/wiki/Calcium-48620. Measurement of the double-beta decay half-life and search for the neutrinoless double-beta decay of 48Ca with the NEMO-3 detector. R. Arnold, C. Augier, AM Bakalyarov, JD Baker, AS Barabash, A. Basharina-Freshville, S. Blondel, S. Blot, M. Bongrand, V. Brudanin, J. Busto, AJ Caffrey, S. Calvez, M. Cascella, C. Cerna, JP Cesar, A. Chapon, E. Chauveau, A. Chopra, D. Duchesneau, D. Durand, V. Egorov, G. Eurin, JJ Evans, L. Fajt, D. Filosofov, R. Flack, X. Garrido, H. G omez, B. Guillon, P. Guzowski, R. Hodak, A. Huber, P. Hubert, C. Hugon, S. Jullian, A. Klimenko, O. Kochetov, SI Konovalov, V. Kovalenko, D. Lalanne, K. Lang, VI Lebedev, Y. Lemi ere, T. Le Noblet, Z. Liptak, XR Liu, P. Loaiza, G. Lutter, F. Mamedov, C. Marquet, F. Mauger, B. Morgan, J. Mott, I. Nemchenok, M. Nomachi, F. Nova, F. Nowacki, H. Ohsumi, RB Pahlka, F. Perrot, F. Piquemal, P. Povinec, P. Pridal, YA Ramachers, A. Remoto, JL Reyss, B. Richards, CL Riddle, E. Rukhadze, NI Rukhadze, R. Saakyan, R. Salazar, X. Sarazin, Yu. Shitov, L. Simard, F. Simkovic, A. Smetana, K. Smolek, A. Smolnikov, S. Soldner-Rembold, B. Soule, I. Stekl, J. Suhonen, CS Sutton, G. Szklarz, J. Thomas, V. Timkin, S. Torre, Vl.I. Tretyak, VI Tretyak, VI Umatov, I. Vanushin, C. Vilela, V. Vorobel, D. Waters, SV Zhukov, and A. Zukauskas, arXiv:1604.01710v3 [hep-ex] 16 Jun 2016,
https://arxiv.org/abs/1604.01710720. Evidence for a Rapid Turnover of Argon in the Lunar Exosphere. Jacob A. Kegerreis, Vincent R. Eke, Richard J. Massey, Simon K. Beaumont, Rick C. Elphic, Luıs F. Teodoro. arXiv:1612.02414v1 [astro-ph.EP] 7 Dec 2016,
https://arxiv.org/abs/1612.02414730. The Evolution and Internal Structure of Jupiter and Saturn with Compositional Gradients, A. Vazan, R. Helled, M. Podolak, A. Kovetz,
https://arxiv.org/abs/1606.01558740. Lunar eclipse induces disturbance in the lunar exosphere, Anil Raghav, Ankush Bhaskar, Virendra Yadav, Nitinkumar Bijewar, Chintamani Pai, Vaibhav Rawoot,
https://arxiv.org/abs/1401.6559750. DUST LEVITATION ABOVE THE LUNAR SURFACE: ROLE OF CHARGE FLUCTUATIONS. Е.V. Rosenfeld, А.V. Zakharov,
https://arxiv.org/abs/1706.09664760. HOW DIELECTRIC BREAKDOWN MAY WEATHER THE LUNAR REGOLITH AND CONTRIBUTE TO THE LUNAR EXOSPHERE. AP Jordan, TJ Stubbs, JK Wilson, PO Hayne, NA Schwadron, HE Spence, NR Izenberg,
https://www.hou.usra.edu/meetings/lpsc2017/pdf/2332.pdf770. PROPERTIES OF THE LUNAR EXOSPHERE DURING THE PERSEID 2009 METEOR SHOWER, Berezhnoy AA, Churyumov KI, Kleshchenok VV, Kozlova EA, Mangano V, Pakhomov YV, Ponomarenko VO, Shevchenko VV, Velikodsky Yu.I,
https://arxiv.org/abs/1404.2075780. SEARCHING FOR LUNAR HORIZON GLOW WITH THE LUNAR ORBITER LASER ALTIMETER. MK Barker, E. Mazarico, DE Smith, X. Sun, MT Zuber, TP McClanahan, GA Neumann, MH Torrence,
https://www.hou.usra.edu/meetings/lpsc2016/pdf/1985.pdf790.
https://phys.org/news/2013-11-plasma-crystal.html830. Растворение натрия в жидком аммиаке, видео:
https://www.youtube.com/watch?v=JefumJFatsw